OUR RESEARCH
Read about our research!
Research Themes and Experimental Approaches
Research in our NSF- and Department of Defense-funded program focuses on the nexus of development, environment and physiology, its manifestation as phenotypic plasticity in both vertebrate and invertebrate animals. In particular, we are investigating the ontogeny of regulation of the physiological systems along the life continuum of eggs, embryos, larvae/fetuses and adults. Our experimental approach is broadly comparative by design. By contrasting and comparing regulatory mechanisms in a developmental series of a wide variety of animals, we can distinguish fundamental developmental processes from those processes that have evolved in the early developmental stages of only particular taxa. We are unabashedly opportunistic in using animals that, according to the Krogh principle (or some would say Bernard principle), help us answer our experimental questions. The earlier in development, the more similar are developing animals, and the more we can identify general principles of physiological development and phenotypic plasticity.
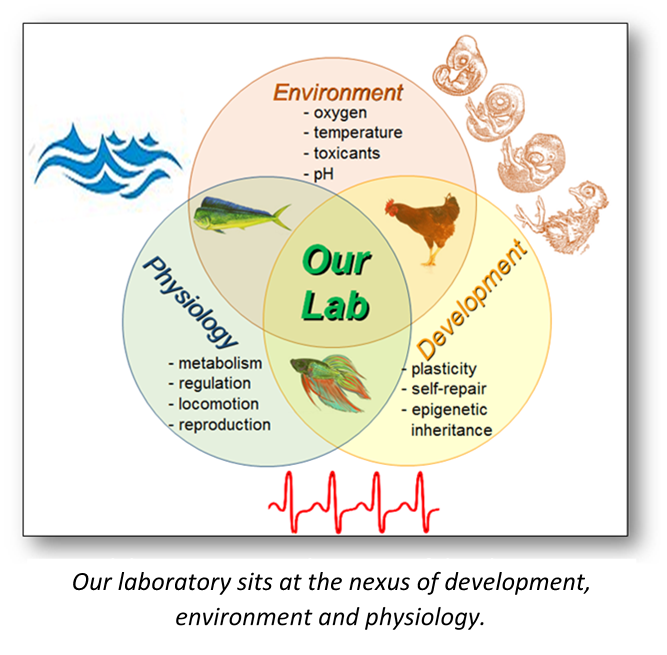
Of special interest is the interplay between environment and development, how the environmental stressors - both natural and anthropogenic - shape emerging phenotype, and how the developing organism can exhibit "self-repair" at the tissue, organ and organismal levels. We typically raise populations of developing animals under challenging environmental conditions to learn if and how the ultimate phenotype is independent of, or linked to, environmental experiences earlier in development.
Typically, we chart the development of basic physiological processes such as the onset of heart beat, development of blood pressure and flow gill or lung ventilation, and osmoregulation. In some experiments, we then determine when and how the cardiovascular and respiratory systems come under neural and endocrine regulation, and how these regulatory processes may change with major developmental events such as hatching (birds, reptiles) or metamorphosis (fishes, amphibians).
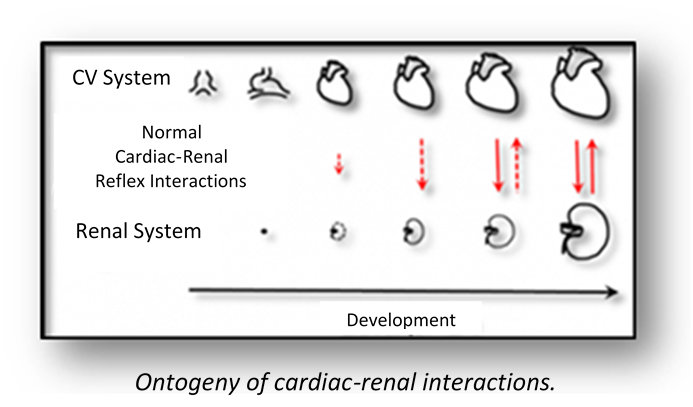
We follow up investigation of basic developmental morphology and further investigate developmental phenotypic plasticity by determining what factors can shape and influence the normal developmental trajectories for physiological regulatory mechanisms. Once the developmental timing of these events is known, we will determine the critical windows during development in which these systems are particularly susceptible to both natural and anthropogenic environmental perturbations of temperature, oxygen availability, acidity, toxicants, etc.
Our experimental approach is broadly comparative by design. By contrasting and comparing regulatory mechanisms in a developmental series of a wide variety of animals, we can distinguish fundamental developmental processes from those processes that have evolved in the early developmental stages of only particular taxa. In this context, our studies of developmental physiology merge with our lab's additional interests in the evolution of physiological processes.
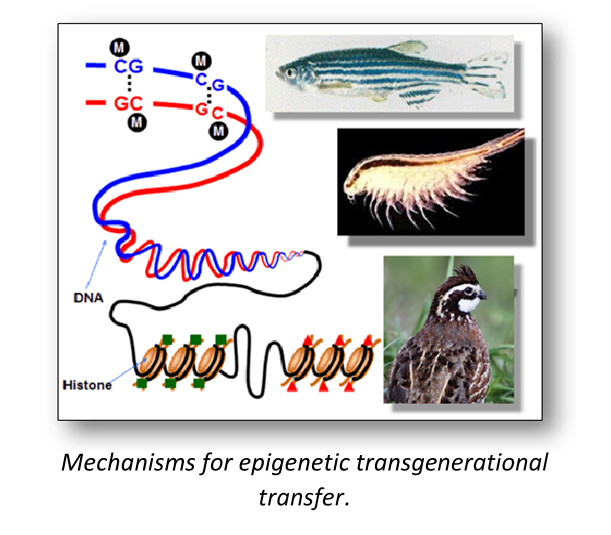
Back to top
Our Animal Models
What is an animal model, when are they used, and what are their limitations? You might find this essay on animal models to be interesting reading on the subject.
The animals listed below are employed in various projects in our laboratory. Why so many different models in our lab, when most labs are "fly labs", "mouse labs", etc? We are interested in the onset of physiological regulation during early development. At these early stages, most of the species below are qualitatively indistinguishable, yet each offers some advantage - transparency, gigantism, life cycle time, etc. At the end of the day, we compare projects, and we can weave a tapestry that is representative of animal development, environment and physiology.
Current Animal Models
Avian Models
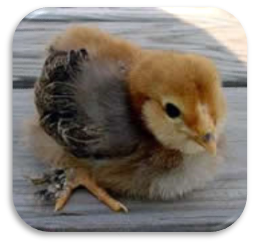 |
- Chicken
(Gallus gallus domesticus)
Serving as one of the main models for physiological development for decades, if not centuries, we are using the chicken embryo to map the ontogeny of cardiovascular, respiratory, and metabolic in chicken embryos under hypoxic and thermal challenge.
|
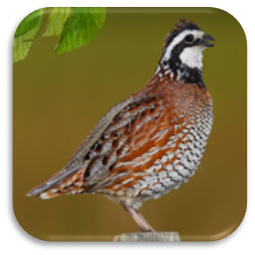 |
- Northern Bobwhite Quail
(Colinus virginianus)
Though the eggs of the quail are quite small, this species represents avian development "in the fast lane", hatching after just 21 days of development. In this regard, it makes for a useful comparison with chickens (21 day incubation) and emus (51 day incubation).
|
Fish Models
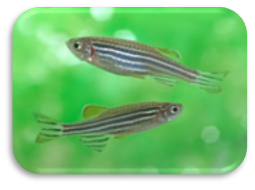 |
- Zebrafish
(Danio rerio)
An increasingly popular model in developmental biology, the zebrafish has also proved very useful in developmental physiology studies. Among other advantages, this teleost fish native to streams in India, is a prolific breeder, producing hundreds of tiny, transparent eggs. Because the heart and circulation can be seen through the translucent body wall for the first 10 days after hatching, we can use optical methods can be used to measure cardiac output, red blood cell velocity, and blood vessel growth and diameter, to name a few. Of course, there is the matter of the embryos weighing only 1/10 mg at hatching, but we have long been on the lunatic fringe in terms of measuring physiological performance in vanishingly small animals...
|
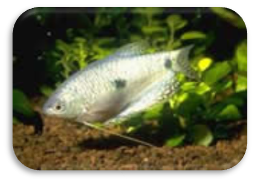 |
- Blue Gourami
(Trichogaster trichopterus)
Very little is known about the developmental physiology of the blue gourami (there have been some papers on the respiratory and metabolic adult physiology), but it has great promise because of its ease of breeding in captivity, its rapid growth, and its obligatory air breathing habitat early in development.
|
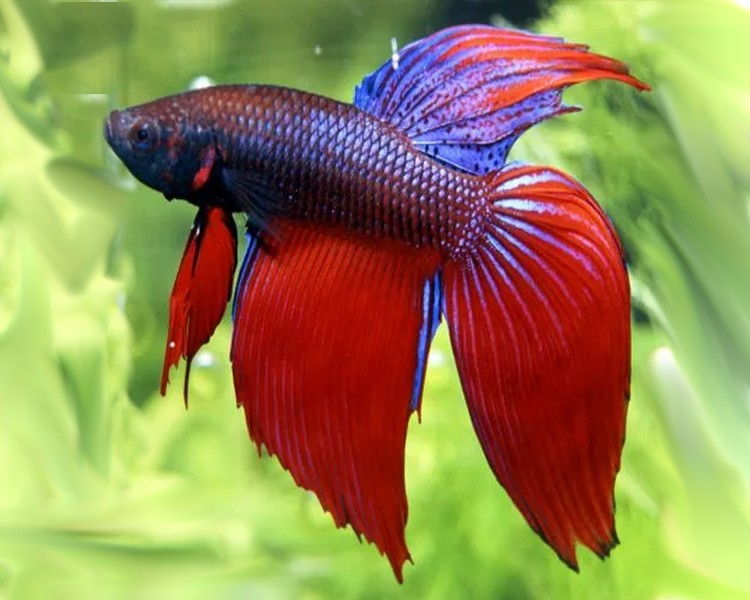 |
- Siamese Fighting Fish
( Beta splendens) )
- Selectively bred by fish enthusiasts around the world, the Siamese Fighting Fish is another air-breathing fish species. The betta is easily bred in captivity and makes a great model for studying the development of air-breathing, particularly in comparison to our other fish model.
|
Invertebrate Models
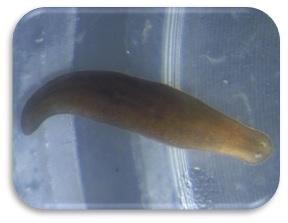 |
- Planaria
(Schmidtea mediterranea)
Planaria are fresh-water, non-parasitic flatworms best known for their ability to regenerate entire individuals from small body fragments, due to a large population of pluripotent stem cells. Schmidtea mediterranea exits in both asexual and sexual strains. Their regenerative ability allows researchers to create clonal lines of both sexes from individual animals. S. mediterranea are widely used in studies of aging, development, regeneration, learning and memory, germ cell specification, stem cell behavior, and cancer.
|
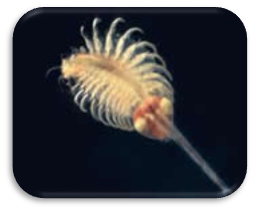 |
- Brine Shrimp
(Artemia franciscana)
The brine shrimp is an excellent model for studying the interactions of environment and phenotype during development. Easily reared in small containers, and with a rapid generation time (8-12 days), the brine shrimp model allows high throughput of multiple environmental stressor X development stage experiments.
|
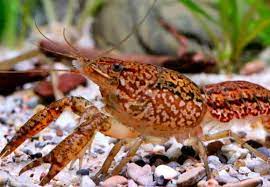 |
- Marbled Crayfish
(Procambarus virginalis)
The Marbled Crafish is a parthenogenicall-reproducing species, such that all individuals are genetically identical clones. This is an extremely useful characteristic for our transgenerational studies, because there is essentially no "noise" from genetic variation.
|
Other Models We Have Used
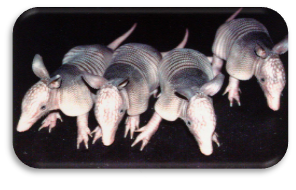 |
- Nine-banded Armadillo
(Dasypsu novemcinctus)
You gotta love the armadillo! Apart from being the only mammal besides man to carry the bacterium causing leprosy, they also show the phenomenon of polyembryony. Shortly after fertilization, the blastula divides into buds that continue to develop as individuals, and each armadillo litter of four is invariably a clonal group with identical genetic make up. We use armadillos to look at between- and within-litter variation in physiological characteristics.
|
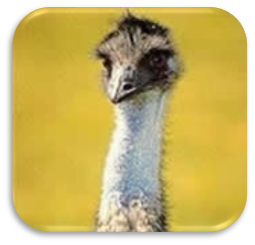 |
- Emu
(Dromaius novaehollandiae)
The emu lays eggs that typically weigh from 650-800 g, about 12-15X greater than a chicken egg. Fortunately, the embryo and all of the extra-embryonic structures are also scaled up by more than an order of magnitude. This makes possible surgical procedures that just can't be done in conventionally studies bird eggs (chicken, quail).
|
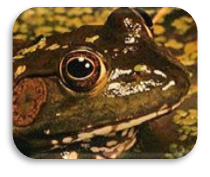 |
- Bullfrog
(Rana catesbeiana)
The bullfrog has long been a standard for developmental physiology, in part because of the large, easily available larvae (tadpoles). In fact, our physiological knowledge of development for the bullfrog rivals that of the chicken. Their wonderful transition from pure water breathers (initially skin, then skin + gills), to combined water and air breathers (skin + gills + lungs) make them an ideal subject for evo-devo studies.
|
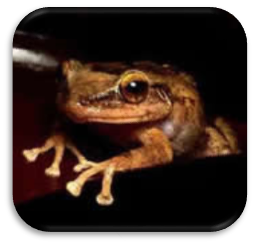 |
- Coqui
(Eleutherodactylus sp.)
The genus Eleutherodactylus is the single largest vertebrate genus, and each of the >450 species is a direct developer. The pea-sized, transparent, terrestrial eggs hatch to reveal a perfectly formed miniature adult. Because the eggs are transparent, we can monitor cardiac function from first beat through "metamorphosis" within the egg to hatching with the adult morph.
|
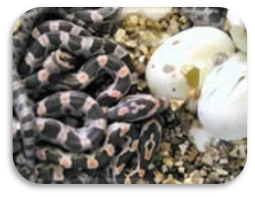 |
- Various Species
The eggs of many reptiles present wonderful opportunities for exploring how environment (specifically, the hydricenvironment) influences development. For example, by adjusting the water potential of the medium in which the eggs are incubated, the water content of the egg - and the blood volume of the embryo - can be manipulated. We have used these animals in the past to determine how the cardiovascular system's baroreceptors begin to function and reach their set-points.
|
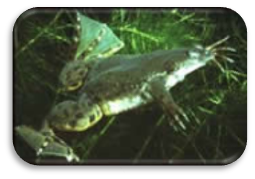 |
- African Clawed Frog
(Xenopus laevis)
Xenopus is the amphibian equivalent of the chicken or the zebrafish with respect to being a prominent developmental model. The normal physiological ontogeny has been well characterized, and our studies now focus on how environmental challenge perturbs normal development, and how the animal in turn copes.
|
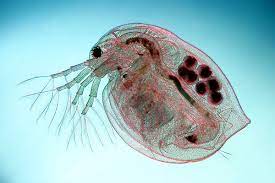 |
- Water Flea (Daphnia magna)
The water flea provides several advantages when looking at transgenerational, epigenetic transfer of phenotype. The life span is short, and clonal populations are readily created and maintained. Add to that the transparency of the adult, and the fact that each female has ~3 broods of offspring make it a powerful model for investigating the "washout" of epigenetic physiological, metabolic and morphologic.
|
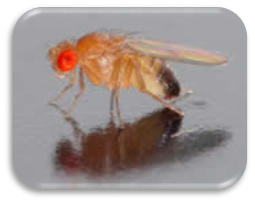 |
- Fruit Fly
(Drosophila melanogaster)
The fruit fly Drosophila melanogaster provides a complex metazoan that nonetheless has a short generation time. This makes it ideal for our studies of physiological and metabolic maternal effects, the non-genetic transmission of characters from parents to offspring.
|
Back to top
Our Methodologies and Expertise
Methodologies and techniques we employee in our laboratory include:
- respirometry, both aerial and aquatic, including microrespirometry of individual eggs/fry/larvae
- blood pressure measurement - conventional and "servo-null" micropressure systems
- blood flow (pulsed Doppler)
- blood analysis - O2, CO2, pH, acid-base, [Hb], P50, hematocrit, etc.
- in situ hybridization, immunohistochemistry
- microscope image analysis for in vivo determination of heart rate, cardiac output, blood velocity, vascular diameters, vascular growth
- microinjection/microwithdrawal for drug injection/blood sampling
- specialized avian egg incubation facilities for hypoxia/hyperoxia
Collaborators
In addition to our lab's close collaboration with the other members of the Developmental Integrative Biology Group at UNT, we also have active, ongoing collaborations at numerous other research institutions, including:
- University of Miami, Florida, USA
- University of Texas, Austin, Texas, USA
- Texas A&M - San Antonio, San Antonio, Texas, USA
- University of California, Riverside
- Universidad Autónoma del Estado de México, Toluca
- Universidad Juarez Autónoma de Tabasco
- AstraZeneca, Gothenburg, Sweden